Upcoming Events!
Past Events
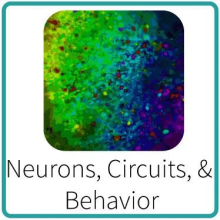
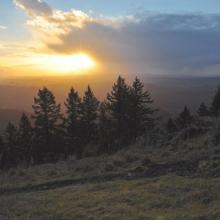
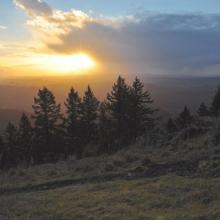
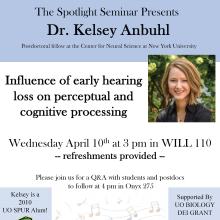
Please join us on Wednesday April 10th for a special seminar with Dr. Kelsey Anbuhl a postdoc at...
Please join us on Wednesday April 10th for a special seminar with Dr. Kelsey Anbuhl a postdoc at the Center for Neural Science at New York University. Kelsey is the selected Spotlight Seminar speaker based on community nominations and the event is supported by a UO Biology DEI grant. She will give a 50 min. research seminar at 3 pm in Willamette 110 on her work investigating the cognitive and perceptual consequences of adolescent hearing loss.
The talk will be targeted at a broad science audience!
At 4 pm in Onyx 275 we will also host a Q&A for trainees with Kelsey and she will talk about her career trajectory and how her own experience with hearing loss inspired her research. See flyer for additional details and we hope to see you there!
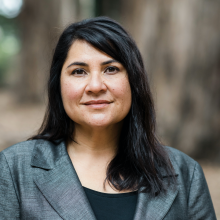
Please note the location change to the Knight Campus Beetham Family Seminar Room and earlier pre...
Please note the location change to the Knight Campus Beetham Family Seminar Room and earlier pre-seminar reception start time of 3:30pm for this week's seminar.
From the sky bridge cross to Knight Campus and take either the stairs of elevator to the first floor (street level). The seminar room will be to the left, and the reception will be just outside of the room.
My lab aims to understand the molecular mechanisms underlying the sensations of itch, touch, and pain. Humans rely on these senses for a broad range of essential behaviors. For example, acute pain acts as a warning signal that alerts us to noxious mechanical, chemical, and thermal stimuli, which can potentially damage tissue. Likewise, itch sensations trigger reflexes that may protect us from disease-carrying insects. Despite these essential protective functions, itch and pain can outlast their usefulness and become chronic diseases. We use cellular physiology, molecular biology, molecular genetics, and behavioral studies to elucidate the mechanisms underlying itch and pain transduction under normal and pathophysiological conditions. This talk will highlight the interactions between the nervous system and immune system that promote chronic itch, pain and inflammation.
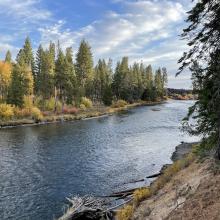
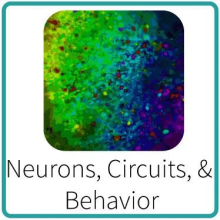
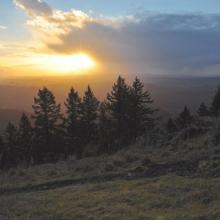
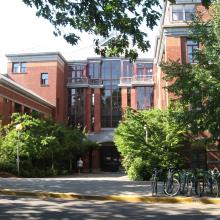
15 minute talks start at 9:00 AM and end at 1:15 PM according to the schedule below
9:00 AM Jackie...
15 minute talks start at 9:00 AM and end at 1:15 PM according to the schedule below
9:00 AM Jackie Kuyat Sylwestrak 9:15 Gonzalo Morales Chaya Sylwestrak 9:30 JoAnna O’Neill Hutchinson (Psych) 9:45 Abbi Koenigsmark Eisen 10:00 Tim Reizis Smear (Psych)
10:15-10:30 BREAK,
10:30 Praves Lamichhane Wehr (Psych) 10:45 Leah Blankenship Jaramillo 11:00 Kasey Drake Huxtable 11:15 Christopher Fields Niell 11:30 Max Horrocks Miller 11:45 Michelle Ortman Mille
12:00-12:15 PM BREAK
12:15 Amberly Buer Cresko 12:30 Katya Podkovyroff Lewis Silva 12:45 Alejandro Santillana Fernandez Hallett 1:00 Elizabeth Diehl Sutherland

15 minute talks run from 9:00 AM to 10:30 AM according to the following schedule:
9:00 AM Maggie...
15 minute talks run from 9:00 AM to 10:30 AM according to the following schedule:
9:00 AM Maggie Barry Garcia (IMB) 9:15 Caroline Crahan Spero (IMB) 9:30 Mary Ruth Shifflett Prell (Chemistry) 9:45 Samara Williams Parthasarathy (Physics) 10:00 Aubrey Mayer Libuda (IMB) 10:15 Laurel Moneysmith Harms (IMB)