Upcoming Events!
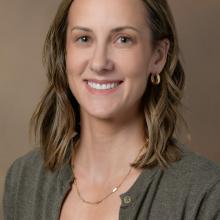
Abstract: Neuroplasticity is an important feature of respiratory control and critical for life, as this allows for adjustments to breathing to fit our metabolic needs across development, disease, injury, and aging. Nicotine is a highly addictive recreational drug that modulates neuronal excitability and plasticity, and the impact of in utero nicotine exposure on the development and function of central networks that control breathing have been extensively studied. Conversely, despite the widespread use of tobacco and nicotine products, we know little about how chronic nicotine exposure impacts breathing control in adulthood. This seminar will highlight some of the ways the Wollman lab studies nicotine-mediated plasticity of respiratory control by giving an overview of past and current research projects including 1) work in neonatal rats showing that in utero nicotine exposure modulates fast-synaptic transmission, indicating a potential mechanism for Sudden Infant Death Syndrome, and 2) Dr. Wollman’s current NIH funded research, which is the first to show the detrimental effects of acute nicotine withdrawal on respiratory chemoreflex control in adult rats.
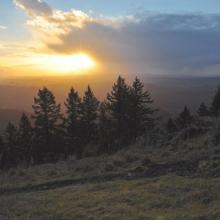
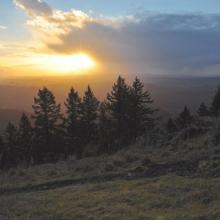
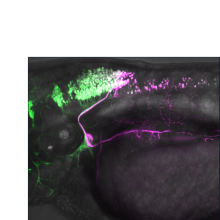
Abstract: During development, the central nervous system establishes precise connections with the body to coordinate organ function. A crucial component of communication between the brain and body is the vagus nerve (cranial nerve X), which innervates multiple organ systems including the heart, lungs and digestive tract to regulate blood pressure, heart rate, respiration and digestion. Despite this important role, the molecular mechanisms guiding the vagus nerve to these organ targets during development remain unknown. We have developed the zebrafish embryo as a powerful model for interrogating vagus nerve development, taking advantage of its optical clarity and genetic accessibility. Using a novel photoconversion-based retrograde axon tracing approach we show that vagal motor neurons (mXns) that project to different organs (e.g. gallbladder, stomach, intestines) are spatially segregated within the hindbrain vagus nucleus. We hypothesize that these distinct mXn "target groups" have distinct molecular identities that guide axon targeting. To test this hypothesis, we have generated a developmental scRNAseq atlas focused on cranial motor neurons and have validated the spatially restricted expression of transcription factors and cell-surface molecules within the vagus motor nucleus. We have generated genetic tools to correlate gene expression with target groups, and performing a reverse mutagenesis screen to test the role of these candidates in topographic map formation, revealing preliminary mXn identity phenotypes. We have also observed that mXn axons contact specific subsets of enteric neurons (ENS) during motor axon pathfinding and have begun testing the role of these contacts in guiding topographic motor targeting.
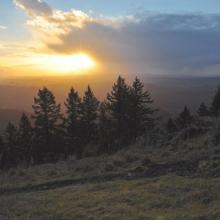
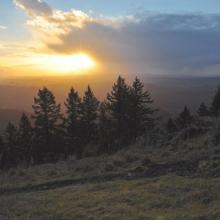
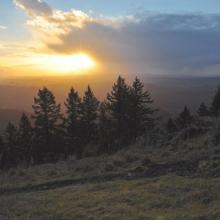
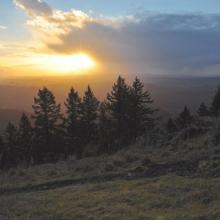
Past Events
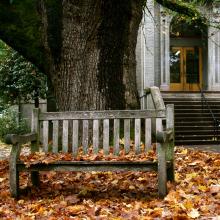
ION Fall Rotation Talks
Tuesday, December 10, 2024
2:30 PM - 3:30 PM in 150 Columbia Hall
- 2:30 PM Joe...
ION Fall Rotation Talks
Tuesday, December 10, 2024
2:30 PM - 3:30 PM in 150 Columbia Hall
- 2:30 PM Joe Wargo - Niell Lab (ION)
- 2:45 PM Danielle Alonzo - Huxtable (Human Phys)
- 3:00 PM Jeremy Guenza-Marcus - McCormick (ION)
- 3:15 PM Brooke Frohock - Gardner (Knight Campus)
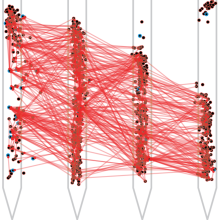
Organisms continually tune their perceptual systems to the features they encounter in their...
Organisms continually tune their perceptual systems to the features they encounter in their environment. We have studied how this experience reorganizes the synaptic connectivity of neurons in the olfactory cortex of the mouse. We developed an approach to measure synaptic connectivity in vivo, training a deep convolutional network to reliably identify monosynaptic connections from the spike-time cross-correlograms of 4.4 million single-unit pairs. This revealed that excitatory piriform neurons that respond similarly to each other are more likely to be connected. We asked whether this like-to-like connectivity was modified by experience but found no effect. Instead, we found a pronounced effect of experience on the connectivity of inhibitory interneurons. Following repeated encounters with a set of odorants, inhibitory neurons that responded differentially to these stimuli both received and formed a high degree of synaptic connections with the cortical network. The experience-dependent organization of inhibitory neuron connectivity was independent of the tuning of either their pre- or their postsynaptic partners. These results suggest the existence of a cell-intrinsic, non-Hebbian plasticity mechanism that depends only on the odor tuning of the inhibitory interneuron. A computational model of this plasticity mechanism predicts that it increases the dimensionality of the entire network’s responses to familiar stimuli, thereby enhancing their discriminability. We confirmed that this network-level property is present in physiological measurements, which showed increased dimensionality and separability of the evoked responses to familiar versus novel odorants. Thus a simple, cell-intrinsic plasticity mechanism acting on inhibitory interneurons may implement a key component of perceptual learning: enhancing an organism’s discrimination of the features particular its environment. [Work with Andrew Fink and Samuel Muscinelli]
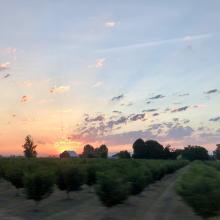
Catch up on the latest ION research. Enjoy delicious food provided by Tasty Thai Kitchen, and great...
Catch up on the latest ION research. Enjoy delicious food provided by Tasty Thai Kitchen, and great conversation provided by your fellow neuroscientists. Bring the family!
For more details & a link to RSVP please see the ion mailing list announcement.
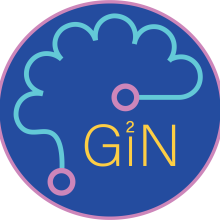
Hello all!
Gender Inclusion in Neuroscience (GiiN) is hosting a Rotation 101 workshop on November...
Hello all!
Gender Inclusion in Neuroscience (GiiN) is hosting a Rotation 101 workshop on November 27th 10-11am in LISB 317! We will be discussing what is expected of a rotation talk and general tips on how to prepare and present. We welcome any and all first years to attend! If you would like to give a practice talk during the workshop, please send an email to ksuzuki@uoregon.edu. Snacks will be provided!
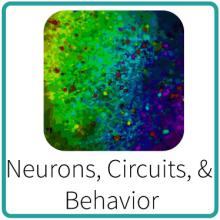
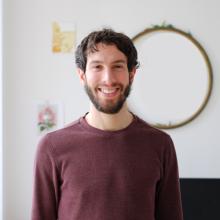
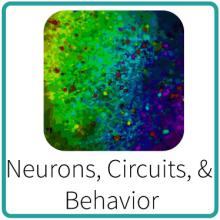
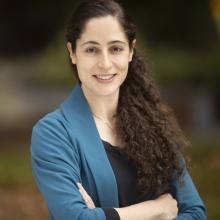
Abstract: For an animal to perform any function, millions of cells in its body furiously interact...
Abstract: For an animal to perform any function, millions of cells in its body furiously interact with each other. Be it a simple computation or a complex behavior, all biological functions involve the concerted activity of many individual units. A theory of function must specify how to bridge different levels of description at different scales. For example, to predict the weather, it is theoretically irrelevant to follow the velocities of every molecule of air. Instead, we use coarser quantities of aggregated motion of many molecules, e.g., pressure fields. Statistical physics provides us with a theoretical framework to specify principled methods to systematically ‘move’ between descriptions of microscale quantities (air molecules) to macroscale ones (pressure fields). Can we hypothesize equivalent frameworks in living systems? How can we use descriptions at the level of cells and their connections to make precise predictions of complex phenomena? My research focuses on the theory, modeling, and analysis required to discover generalizable forms of scale bridging across species and behavioral functions. In this talk, I will present lines of previous and ongoing research that highlight the potential of this vision. I shall focus on two seemingly very different systems: mouse brain neural activity patterns, and octopus skin cells activity patterns. In the mouse, we reveal striking scaling behavior and hallmarks of a renormalization group- like fixed point governing the system. In the octopus, camouflage skin pattern activity is reliably confined to a (quasi-) defined dynamical space. Finally, I will touch upon the benefits of comparing across animals to extract principles of multiscale function in biological systems, and discuss potential avenues of investigation that could allow us to decipher how macroscale properties, such as memory or camouflage, emerge from microscale level activity of individual cells.
Leenoy Meshulam is a theory fellow at the University of Washington in Seattle, and a Burroughs Wellcome CASI awardee. Her research interests are at the intersection of physics, biology, and neuroscience. Dr. Meshulam received her Ph.D. from Princeton University in 2018. Prior to that, she completed her M.Sc. summa cum laude at Tel Aviv University in 2012 and graduated the Adi Lautman Interdisciplinary Honors Program for Outstanding Students.
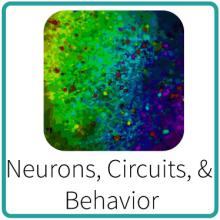
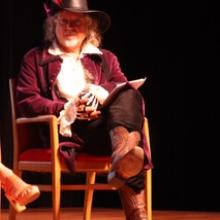
Dr. Bower is a computational neurobiologist who received his PhD in neurophysiology at the...
Dr. Bower is a computational neurobiologist who received his PhD in neurophysiology at the University of Wisconsin Madison, and completed postdoctoral studies at New York University and the Marine Biological Laboratory in Woods Hole, Massachusetts. His first faculty appointment was at the California Institute of Technology (Caltech), where over 17 years he helped found the first graduate program in computational neuroscience. Subsequent to Caltech he was a professor of Computational Neuroscience within the University of Texas System.
In addition to model and experimental based research on the olfactory system and cerebellum, Dr. Bower’s laboratory has also been involved in numerous infrastructure projects including constructing GENESIS, one of the first simulation platforms supporting detailed models of neuronal structures. Dr. Bower also founded the annual international conference on Computational Neuroscience, the first summer courses in computational neuroscience in the United States, Europe and Latin America, and the Journal of Computational Neuroscience. Dr. Bower was also involved in the re-emergence of neural networks, co- organizing the first NIPS meeting, (recently re-named NEurIPS), which has become one of the largest and most important annual meetings in Machine Learning. Dr. Bower is the author of many scientific research articles and has edited and written numerous books. While he is now officially retired, he remains an affiliate faculty member at Southern Oregon University and the University of Hertfordshire in the UK.
In addition to his work in science, Dr. Bower has also had a long-standing interest and involvement in science education and digital-based educational innovation. While a professor at Caltech, Dr. Bower founded and for 17 years co-directed the Caltech Precollege Science Initiative (CAPSI) a multi-million-dollar hands-on science education outreach program to majority minority school districts in the State of California. While supporting the introduction of hands-on science learning in public schools, Dr. Bower’s efforts in CAPSI also explored the use of digital technology in support of science learning. In 1998 Dr. Bower founded Numedeon Incorporated, which in 1999 launched Whyville.net the first game-based learning virtual world. Whyville now has over 8.5 million cumulative registered users worldwide. In 2014, Dr. Bower founded the company Virtual Worlds IP, intended to support the wider application of the simulation-game-based virtual world learning engine developed using Whyville. That technology is protected by a core and foundation patent in on-line virtual worlds (US patent # 7,925,703).